- Magazine
- Material science insights
- Silicon versus silicone: What’s the difference?
Silicon versus silicone: What’s the difference?
With such similar names, you could be forgiven for thinking that silicon and silicone must also have similar properties. But in fact the opposite is the case. In this article, we examine the chemical structures of silicon and silicones, the effect this has on their properties, and what this means for their applications.
Introducing silicon and silicones
In many languages, simply adding a letter to a word can result in a very different meaning – with good examples in English being spin and spine, tub and tube, or tap and tape.
Frustratingly for those seeking to understand materials science, the same is true of two commonplace substances, silicon and silicone. As a result, these two materials are often confused, when in fact they have very different structures, properties and applications. So our aim in this article will be to make everything clearer!
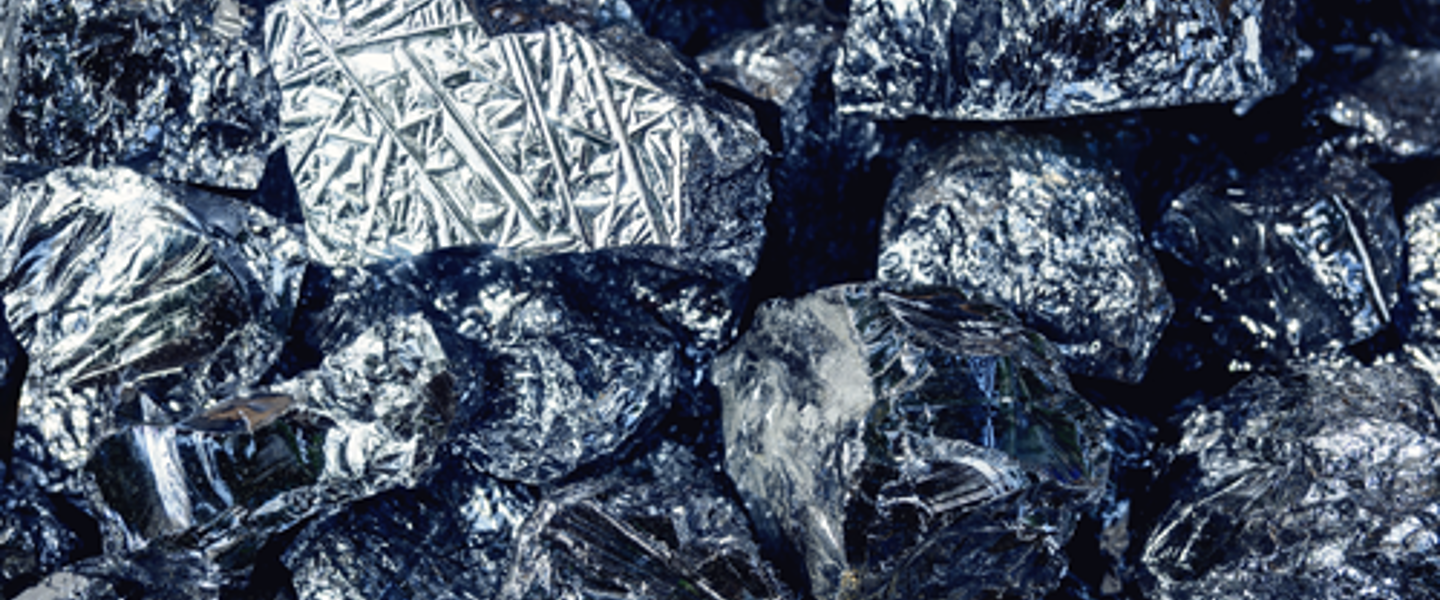
Silicon and silicones – The basics
The first thing to do is to be clear about the structural difference between silicon and silicones.
Silicon is a chemical element, which (when pure) contains just silicon atoms. When the element is in its crystalline form, these silicon atoms are arranged in an infinite network, with each atom connected tetrahedrally to four others (very much like diamond).
The structure of silicon
Silicones are a large group of synthetic polymers containing equal amounts of silicon and oxygen, and variable amounts of carbon, hydrogen, and sometimes other elements.
The silicon and oxygen alternate in a long polymer chain, with everything else branching off from the silicon as ‘substituents’.
The structure of the simplest silicone polymer, poly(dimethylsiloxane), showing how the Si–O linkages in the polymer chain provide the flexibility that is crucial to so many applications.
Why are the properties of silicones so different from silicon?
As is clear from the diagrams above, the structures of silicon and silicones are very different, which has a major influence on their properties.
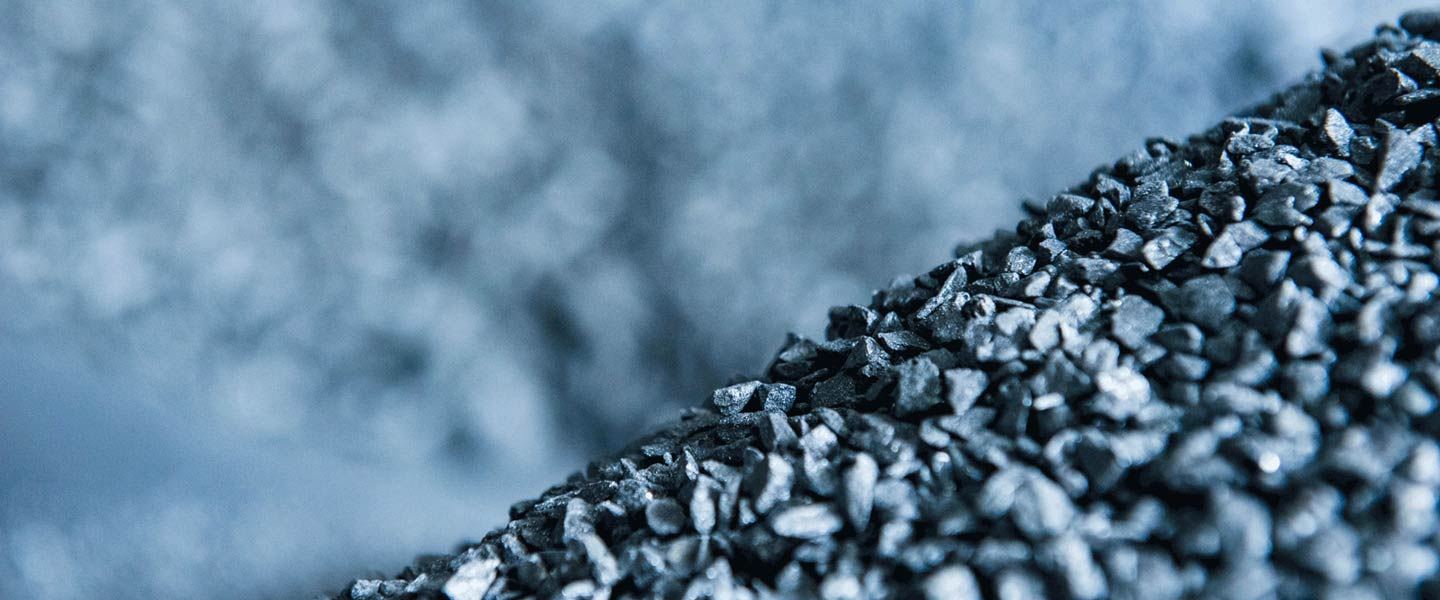
Silicon 101 - The properties of silicon
Firstly, the polymeric nature of silicones means that the chains are flexible, unlike the rigid network of atoms in crystalline silicon. This makes silicones generally soft and flexible. In fact, this property is enhanced further by the presence of oxygen atoms within the chain. Oxygen can only have two bonds, and so it does not carry any substituents, meaning the Si–O–Si linkages are relatively free to rotate. This means that silicone molecules can easily slide over each other, further reducing their viscosity.
This flexibility of silicones also makes them thermally insulating, because it means that they strongly absorb thermal vibrations (phonons). In contrast, silicon has a very rigid structure, meaning the vibrations are easily transmitted through the crystal, and in fact making pure silicon an unusually good conductor of heat.
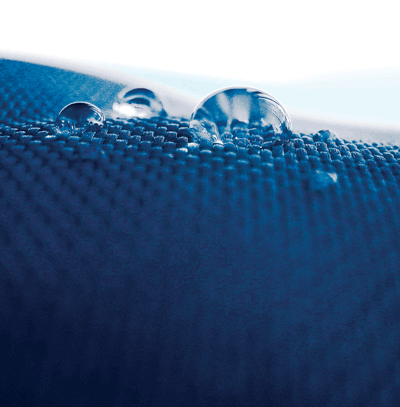
Other elements are conspicuously absent from the structure of pure silicon – unlike silicones, which have large numbers of carbon-containing groups. In particular, the presence of non-polar alkyl groups such as methyl (CH3) or phenyl (C6H5) on the outside of the silicone chains means that they have weak interactions with water and other polar molecules, making them hydrophobic and chemically inert (and also biocompatible). Silicon is also chemically unreactive, but for a rather different reason – not only is its crystal lattice difficult to break apart, but a single-atomic layer of oxygen on the surface of silicon stops it from reacting in the first place.
The next thing to consider is that the silicon–oxygen bonds in the ‘backbone’ of silicones are pretty strong. This makes silicones resistant to thermal decomposition.
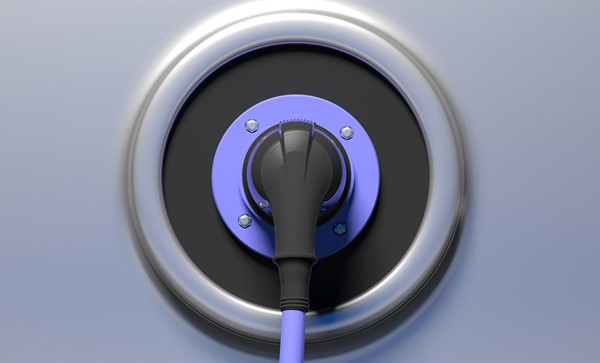
Silicon is also thermally resistant, but once again this is because of the strength of its lattice (the bonds themselves are fairly weak, there’s just lots of them).
Last but not least, the electrons within silicones are tightly bound within covalent bonds – so they cannot move around and conduct electricity, making silicones electrically insulating. The situation with silicon is somewhat different, because in this case the application of heat can make electrons move through the lattice – a phenomenon that increases as the temperature is increased, and which is a hallmark of semiconductors.
How can the properties of silicon and silicones be tailored?
Both silicon and silicones are remarkably versatile materials, in the sense that their properties can be fine-tuned to the application. However, how that’s done is different between silicon and silicones, so here’s a quick run-through of the options.
Tailoring the properties of silicon
You might think that as a pure element, there’s not much that can be done to modify the properties of silicon. But there’s actually quite a bit of choice:
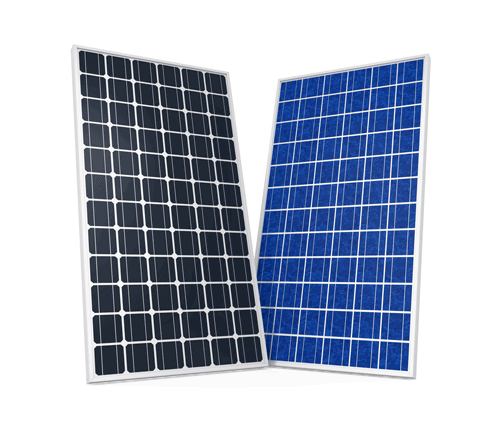
- Adding dopants: When pure, silicon is a weak electrical semiconductor, but this useful property can be greatly enhanced by adding small quantities of other elements as dopants – which is essential for applications in electronics.
- Tailoring the particle size: Silicon can be provided in various sizes. Large blocks of monocrystalline silicon are the starting point for the manufacture of integrated circuits, while ‘micronized silicon’ with particle sizes of 75 µm and below is valuable for alloy production and further processing in industrial applications.
- Adjusting the crystallinity: The atoms in silicon need not have a completely ordered arrangement. Amorphous silicon (where the silicon atoms have no long-range ordering) can be applied in thin layers for flexibility; polycrystalline silicon (with lots of small crystallites joined together) provides good semiconducting properties for use in solar cells; and monocrystalline silicon (a single completely ordered crystal) is needed for the most demanding applications in electronics.
Tailoring the properties of silicones
Like many other polymers, there are lots of ways to modify the properties of silicones to suit the application. These are some of the most important:
- Adjusting the chain lengths: The molecular weight of a silicone polymer is a key factor in its properties – short chains will generally produce soft solids or even oils, while long chains are more easily entangled, producing elastomers with rubber-like properties.
- Modifying the chain branching: Silicone chains need not be completely linear. Introducing branching points within the chains increases the degree of chain entanglement, increasing the viscosity and producing hard-wearing resins.
- Changing the substituents: The poly(dimethylsiloxane) illustrated earlier is the simplest and most widely-used silicone polymer, but the two methyl groups on each silicon atom can be replaced with essentially anything else, to give a ‘functionalized’ product. For example, chemical inertness, temperature resistance and UV resistance can be enhanced by using phenyl groups, while silicones can be made more reactive by using amino groups.
- Adjusting the degree of cross-linking: Making links between silicone polymer chains (or between silicone and other components) effectively ties them together, pulling them back together if they are stretched. The links are made by introducing special reactive groups during the early stages of silicone synthesis, which allows them to be later cross-linked when needed using a catalyst, alkali or heat. In this way, silicone elastomers can be produced.
- Using additives: Silicones can also be modified by adding non-reactive components. For example, adding a lithium soap thickens the consistency to make a grease; adding finely-divided silica produces antifoaming agents (the silica crystals basically pop any bubbles); and adding fillers can improve hardness and other mechanical properties.
The applications of silicon and silicones
With their vastly different structures and properties, and the numerous ways in which the basic materials can be modified, both silicon (and its alloys) and silicones have a wide array of applications:
Some of the main uses of silicon :
Elemental silicon
- Integrated circuits (monocrystalline silicon)
- Solar cells (polycrystalline silicon (or strictly speaking, multicrystalline silicon) or monocrystalline silicon)
- Lithium-ion batteries (various forms)
- Plasma sputtering targets (micronized silicon)
- Pyrotechnic ignition compositions (micronized silicon)
- Chemical production (silicon powder forms)
- Advanced Technical Ceramics
Silicon alloys
- Steel production (iron–silicon)
- Cast iron production (iron–magnesium–silicon)
- Castings for engine parts (aluminium–silicon)
- Low-temperature brazeable joints (aluminium–copper–silicon)
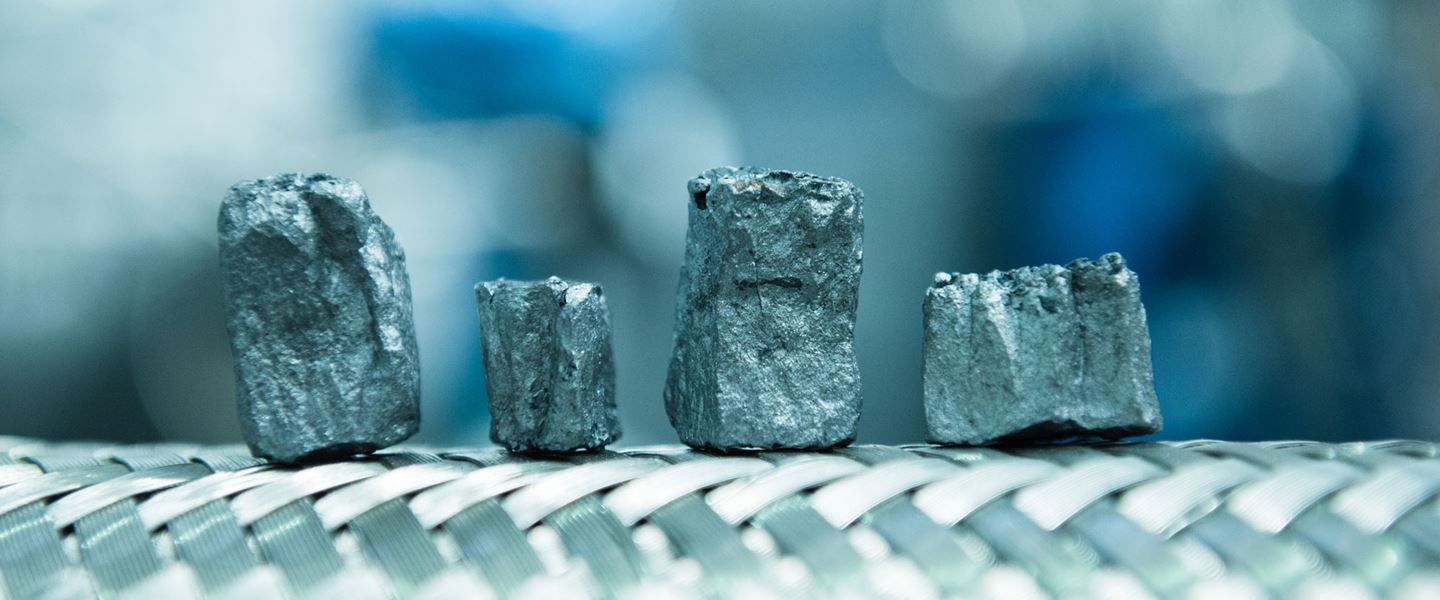
Silicon 101 - What is silicon used for?
Some of the main uses of silicones
- Adhesives and sealants
- Electrical insulation (including high-voltage lines)
- Non-stick kitchenware and utensils
- Medical/dental devices and implants
- Keypads
- Lubricants and release agents
- Antifoaming agents (when compounded with silica)
- Dielectrics
- Engineering fluids
- Paint additives
- Facial tissues, cosmetics and homecare products
- Pharmaceuticals and other medical applications.
- Release agents and lubricants for plastic products
- Car polishes and household cleaners
- Tactile/water-repellent textile finishes
- Water-repellent agricultural/building products
- Lubricants and antistatic agents in printing and papermaking.
- Heat-resistant paint binders
- Electrical insulation
- High-temperature non-stick coatings
- Water-repellent construction treatments
- Paper/film release coatings.
- Oil and gas extraction equipment
- Water and effluent treatment
- Laboratory equipment
- Lubricants
- O-Ring sealants.
Silicone pastes
- Lubricants and installation aids
- Sealant aids for moveable parts
- High-temperature release agents.
Conclusion: A world of applications
It should be clear from this article that there’s not only a great deal of difference between the structures and properties of silicon and silicones, but that there’s also great variety in their applications too.
From solar cells to sealants, and from castings to coatings, silicon and silicone play a major role in our lives… and so we’d say it’s definitely worth taking care with that letter ‘e’!